Category
- Products
- FAQ
- Contact us
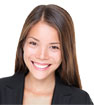
Frequency inverter manufacturers
Frequency Inverter Harmonics Solutions
Frequency inverters can be both a source and a victim of poor power quality.
Frequency Inverters as Victim Loads
Although frequency inverters are usually depicted as the culprit in the PQ scenario, there are ways in which they can be a victim load as well.
Capacitor switching transients
High-energy (relatively low frequency) transients that are characteristic of utility capacitor switching can pass through the service transformer, feeders, and converter front-end of the frequency inverter directly to the dc link bus, where it will often cause a dc link overvoltage trip. Input diodes could also be blown out by these transients.
Voltage distortion
If high-voltage distortion shows up as excessive flat-topping, it will prevent dc link capacitors from charging fully and will diminish the ride-through capability of the frequency inverter. Thus a voltage sag which would not normally affect a frequency inverter will cause the frequency inverter to trip on undervoltage.
Improper grounding will affect the internal control circuits of the frequency inverter, with unpredictable results.
Frequency Inverters as Culprit Loads
A frequency inverter can definitely be a "culprit load" and have a major impact on system PQ. But before we talk of problems, let's put in a good word for the positive effects of frequency inverters on PQ. First of all, they offer built-in soft-start capabilities. This means there will be no inrush current and no voltage sag effect on the rest of the system. Secondly, if the frequency inverter is of the PWM type, with a diode converter front-end, the Displacement Power Factor is high (commonly > 95 % at rated load) and more or less constant throughout the range. This means that frequency inverters can reduce energy usage and correct for displacement power factor at the same time. It's a good thing too, because frequency inverters and power factor correction capacitors don't mix. Caps are vulnerable to the higher frequency harmonic currents generated by frequency inverters, since their impedance decreases as frequency increases.
The type of frequency inverter has a major impact on the PQ symptoms, because of the different converter designs (converters or rectifiers turn ac to dc and are the first stage of the frequency inverter). There are two major types of converter design.
SCR Convertor with Voltage Source Inverter/Variable Voltage Inverter (VSI/VVI)
Commonly called six-step frequency inverters, they use SCRs (Silicon - Controlled Rectifiers) in their converter front-ends (the following discussion applies to CSI, Current Source Inverter frequency inverters, which also use SCRs). VSI and CSI frequency inverter designs tended to be applied on larger frequency inverters (> 100 HP). SCR converters control the dc link voltage by switching on (or "gating") current flow for a portion of the applied sine wave and switching off at the zero crossing points. Unlike diodes, SCRs require control circuits for gate firing.
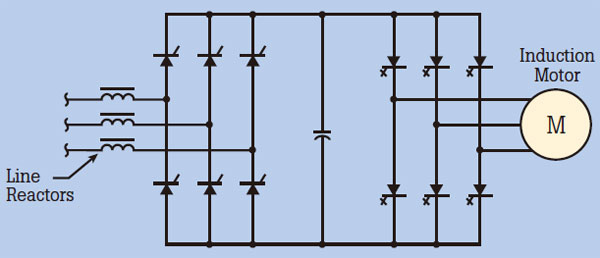
For the SCR converter, there are three main issues that affect line-side PQ:
The other and more common converter design uses diodes and is used in the PWM frequency inverter. The diodes require no switching control circuitry. One of the main trends in the industry has been the proliferation of PWM frequency inverters, mainly due to the continued development of fast-switching, efficient IGBTs (Insulated Gate Bipolar Transistors) used in the inverter section of the frequency inverter (inverters turn dc to ac). For all practical purposes, PWM frequency inverters are the industry standard.
For the diode converter, the main PQ issue is harmonics. The actual harmonic orders being generated depend on the number of diodes in the front end. For three-phase conversion, a minimum set of six diodes is required. This "six-pulse" converter will generate 5th and 7th harmonics. If a 12-pulse converter were used, the 11th and 13th harmonics will be generated instead of the 5th and 6th - and, very importantly, for the same load, the amplitude of the 11th and 13th would be considerably less than the 5th and 6th. Therefore, the THD would be less. The vast majority of frequency inverters, however, are six-pulse PWM style, which is one reason we see so much 5th harmonic on the system.
Harmonics Solutions
There are a number of solutions to mitigating frequency inverter generated harmonics:
Harmonic trap filters
These are typically LC networks connected in parallel at the source of the harmonics (in other words, at the frequency inverter input). They are tuned to just below the 5th harmonic (typically 280 Hz) and will tend to sink both 5th and much of the 7th harmonic. Obviously, they must be sized to the harmonic-generating load.
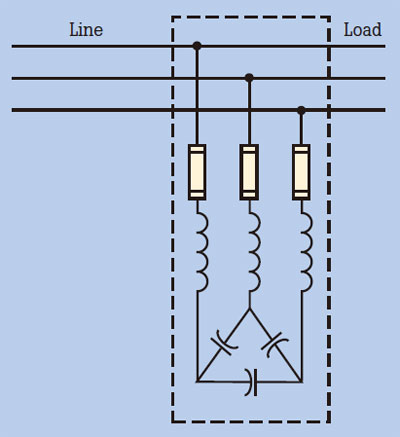
Phase-shift transformers
This can be as simple as a delta wye transformer feeding one frequency inverter(s) and a delta-delta feeding another frequency inverter(s). There is a 30 degree phase shift effect between these two configurations, which effectively results in cancellation of harmonics at the closest upstream PCC (Point of Common Coupling). The cancellation effect is optimal when both loads are more or less equal.
12-pulse converter
If the delta-wye/delta-delta are packaged together (delta primary, delta and wye secondary) and each secondary feeds one of two paralleled six-pulse converters, a 12-pulse front-end is created with all the benefits mentioned above. 18-pulse designs are also available. Because of the extra cost, this type of solution tends to only get used on high HP loads.
Active filters
This relatively new technology is based on an elegant concept - using power electronics to solve the problems created by power electronics. It senses the instantaneous ac sine wave; it then actively cancels the harmonics it detects by generating equal and opposite polarity harmonics, thus recreating the sine wave. Commercial packages might provide voltage regulation as well.
Active power factor correction
Another recent solution is for manufacturers to offer converter front ends using fast switching technology that generates a minimum amount of harmonics and has near unity power factor (both Total power factor and displacement power factor). There is room for discussion on which approach to harmonic mitigation might prove most effective and economical in a particular situation. However, what is often overlooked by the end-user, and what should be clear from the information in this section, is that the total cost of a frequency inverter system should include both the cost of the frequency inverter itself and the harmonic mitigation (whether part of the frequency inverter or installed separately).
Power system resonance
Is it possible to install "Power Factor Correction Capacitors" and have power factor get worse? It certainly is, and a starting place to understanding this puzzle lies in the distinction between displacement power factor (DPF) and Total Power Factor (PF). The penalty for not understanding the difference can be blown capacitors and wasted investment.
Total power factor and Displacement power factor are the same in one basic sense: they are the ratio of Real Power to Apparent Power, or Watts to VA. Displacement power factor is the classic concept of power factor. It can be considered as the power factor at the fundamental frequency. Total Power Factor, abbreviated to Power Factor (PF), now includes the effects of fundamental and of harmonic currents (it is also referred to as True power factor or Distortion power factor, Below figure). It follows that with the presence of harmonics, power factor is always lower than displacement power factor and is also a more accurate description of total system efficiency than displacement power factor alone.
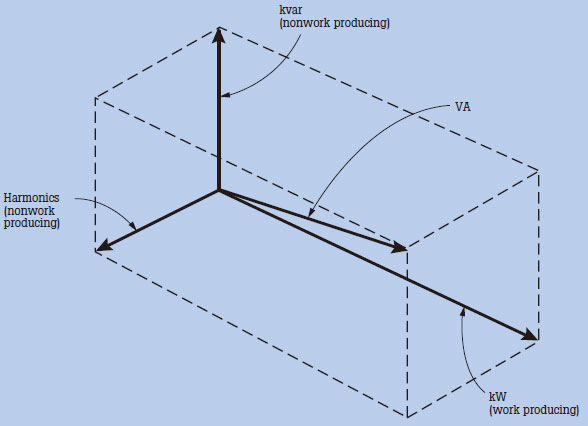
Strictly speaking, the term "Power Factor" refers to Total power factor, but in practice can also be used to refer to displacement power factor. Needless to say, this introduces some confusion into discussions of power factor. You have to be clear which one you’re talking about.
Displacement power factor
Lower displacement power factor is caused by motor loads which introduce the need for Reactive Power (Volt-Amp Reactive or VARs). The system has to have the capacity, measured in Volt-Amps (VA) to supply both VARs and Watts. The more VARs needed, the larger the VA requirement and the smaller the displacement power factor. The cost of VARs is accounted for in a power factor penalty charge. Utilities often levy additional charges for displacement power factor below a certain level; the actual number varies widely, but typical numbers are 0.90 to 0.95.
To reduce VARs caused by motor loads, power factor correction capacitors are installed. Upstream system capacity, both in the plant and at the utility level, is released and available for other uses.
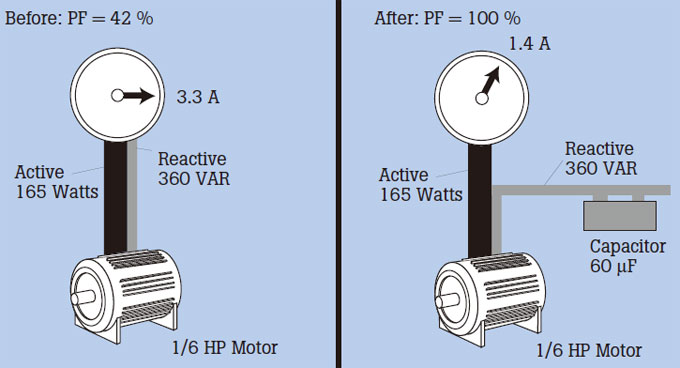
Historically, this has been the gist of the power factor story: a relatively well-known problem with a relatively straightforward solution.
Harmonics and capacitors
Harmonics have had a dramatic impact on our approach to Power Factor correction. The motor and capacitor loads described above are all linear and for all practical purposes generate no harmonics. Non-linear loads such as frequency inverter, on the other hand, do generate harmonic currents.
Take a plant which is step-by-step putting frequency inverters on its motor loads. Frequency inverters generate significant harmonic currents (5th and 7th on six pulse converter frequency inverters). Suddenly the fuses on existing power factor correction caps start blowing. Since these are three-phase caps, only one of the three fuses might blow. Now you’ve got unbalanced currents, possibly unbalanced voltages. The electrician replaces the fuses. They blow again. He puts in larger fuses. Now the fuses survive, but the capacitor blows. He replaces the capacitor. Same thing happens. What’s going on? Harmonics are higher frequency currents. The higher the frequency, the lower the impedance of a cap (XC = 1/2πfC). The cap acts like a sink for harmonic currents.
Power system resonance
In a worst-case scenario, the inductive reactance (XL) of the transformer and the capacitive reactance (XC) of the power factor correction cap form a parallel resonant circuit: XL = XC at a resonant frequency which is the same as or close to a harmonic frequency. The harmonic current generated by the load excites the circuit into oscillation. Currents then circulate within this circuit which are many times greater than the exciting current. This so-called "tank circuit" can severely damage equipment, and it will also cause a drop in power factor. Perversely, this resonant condition often appears only when the system is lightly loaded, because the damping effect of resistive loads is removed. In other words, we have what the audio buffs call a "high Q" circuit.
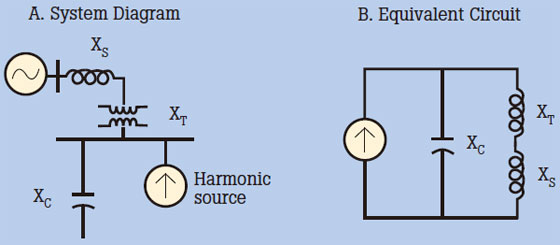
Imagine coming to work on a Monday and seeing the insulation on your cables melted off. How can this happen over a weekend when there was hardly any load on the system? Has Ohm's Law been overruled? Not quite. Your power system just spent the weekend tanked out on the Harmonics. It was quite a party, but now comes the clean-up.
Start with harmonics mitigation
The correct solution path starts with measuring and mitigating the harmonics generated by the frequency inverters. Harmonic trap filters would generally be called for. These trap filters are installed locally on the line side of the frequency inverter. Their effect is very much like the traditional power factor correction cap, in two senses: they reduce displacement power factor as well as power factor, and also they localize the circulation of the problem harmonics (generally the 5th). Harmonics mitigation and traditional displacement power factor correction should be addressed as one systems issue. In other words, manage Total power factor, not just displacement power factor.
Frequency Inverters as Victim Loads
Although frequency inverters are usually depicted as the culprit in the PQ scenario, there are ways in which they can be a victim load as well.
Capacitor switching transients
High-energy (relatively low frequency) transients that are characteristic of utility capacitor switching can pass through the service transformer, feeders, and converter front-end of the frequency inverter directly to the dc link bus, where it will often cause a dc link overvoltage trip. Input diodes could also be blown out by these transients.
Voltage distortion
If high-voltage distortion shows up as excessive flat-topping, it will prevent dc link capacitors from charging fully and will diminish the ride-through capability of the frequency inverter. Thus a voltage sag which would not normally affect a frequency inverter will cause the frequency inverter to trip on undervoltage.
Improper grounding will affect the internal control circuits of the frequency inverter, with unpredictable results.
Frequency Inverters as Culprit Loads
A frequency inverter can definitely be a "culprit load" and have a major impact on system PQ. But before we talk of problems, let's put in a good word for the positive effects of frequency inverters on PQ. First of all, they offer built-in soft-start capabilities. This means there will be no inrush current and no voltage sag effect on the rest of the system. Secondly, if the frequency inverter is of the PWM type, with a diode converter front-end, the Displacement Power Factor is high (commonly > 95 % at rated load) and more or less constant throughout the range. This means that frequency inverters can reduce energy usage and correct for displacement power factor at the same time. It's a good thing too, because frequency inverters and power factor correction capacitors don't mix. Caps are vulnerable to the higher frequency harmonic currents generated by frequency inverters, since their impedance decreases as frequency increases.
The type of frequency inverter has a major impact on the PQ symptoms, because of the different converter designs (converters or rectifiers turn ac to dc and are the first stage of the frequency inverter). There are two major types of converter design.
SCR Convertor with Voltage Source Inverter/Variable Voltage Inverter (VSI/VVI)
Commonly called six-step frequency inverters, they use SCRs (Silicon - Controlled Rectifiers) in their converter front-ends (the following discussion applies to CSI, Current Source Inverter frequency inverters, which also use SCRs). VSI and CSI frequency inverter designs tended to be applied on larger frequency inverters (> 100 HP). SCR converters control the dc link voltage by switching on (or "gating") current flow for a portion of the applied sine wave and switching off at the zero crossing points. Unlike diodes, SCRs require control circuits for gate firing.
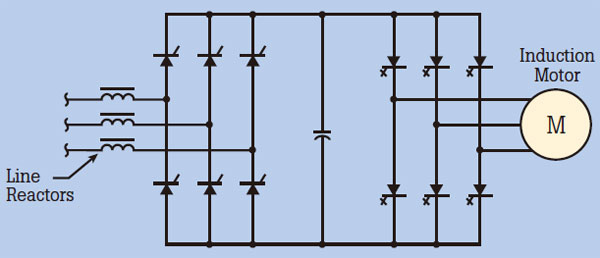
For the SCR converter, there are three main issues that affect line-side PQ:
- Commutation notches. SCR switching or commutation is such that there are brief moments when two phases will both be "ON." This causes what is in effect a momentary short circuit that tends to collapse the line voltage. This shows up as "notches" on the voltage waveform. These notches cause both high VTHD and transients. The solution is to place a reactor coil or isolation transformer in series with the frequency inverter's front end to clean up both problems.
- Displacement Power Factor declines as frequency inverter speed decreases. This is not as serious a problem as it sounds, because the power requirement of the drive-motor-load decreases even more.
- Harmonic currents, typically the 5th and 7th, are generated by VSI frequency inverters.
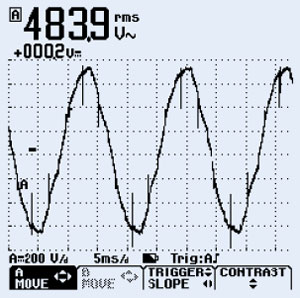
For the diode converter, the main PQ issue is harmonics. The actual harmonic orders being generated depend on the number of diodes in the front end. For three-phase conversion, a minimum set of six diodes is required. This "six-pulse" converter will generate 5th and 7th harmonics. If a 12-pulse converter were used, the 11th and 13th harmonics will be generated instead of the 5th and 6th - and, very importantly, for the same load, the amplitude of the 11th and 13th would be considerably less than the 5th and 6th. Therefore, the THD would be less. The vast majority of frequency inverters, however, are six-pulse PWM style, which is one reason we see so much 5th harmonic on the system.
Harmonics Solutions
There are a number of solutions to mitigating frequency inverter generated harmonics:
Harmonic trap filters
These are typically LC networks connected in parallel at the source of the harmonics (in other words, at the frequency inverter input). They are tuned to just below the 5th harmonic (typically 280 Hz) and will tend to sink both 5th and much of the 7th harmonic. Obviously, they must be sized to the harmonic-generating load.
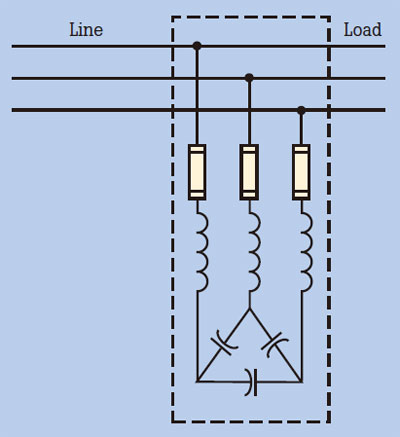
Phase-shift transformers
This can be as simple as a delta wye transformer feeding one frequency inverter(s) and a delta-delta feeding another frequency inverter(s). There is a 30 degree phase shift effect between these two configurations, which effectively results in cancellation of harmonics at the closest upstream PCC (Point of Common Coupling). The cancellation effect is optimal when both loads are more or less equal.
12-pulse converter
If the delta-wye/delta-delta are packaged together (delta primary, delta and wye secondary) and each secondary feeds one of two paralleled six-pulse converters, a 12-pulse front-end is created with all the benefits mentioned above. 18-pulse designs are also available. Because of the extra cost, this type of solution tends to only get used on high HP loads.
Active filters
This relatively new technology is based on an elegant concept - using power electronics to solve the problems created by power electronics. It senses the instantaneous ac sine wave; it then actively cancels the harmonics it detects by generating equal and opposite polarity harmonics, thus recreating the sine wave. Commercial packages might provide voltage regulation as well.
Active power factor correction
Another recent solution is for manufacturers to offer converter front ends using fast switching technology that generates a minimum amount of harmonics and has near unity power factor (both Total power factor and displacement power factor). There is room for discussion on which approach to harmonic mitigation might prove most effective and economical in a particular situation. However, what is often overlooked by the end-user, and what should be clear from the information in this section, is that the total cost of a frequency inverter system should include both the cost of the frequency inverter itself and the harmonic mitigation (whether part of the frequency inverter or installed separately).
Power system resonance
Is it possible to install "Power Factor Correction Capacitors" and have power factor get worse? It certainly is, and a starting place to understanding this puzzle lies in the distinction between displacement power factor (DPF) and Total Power Factor (PF). The penalty for not understanding the difference can be blown capacitors and wasted investment.
Total power factor and Displacement power factor are the same in one basic sense: they are the ratio of Real Power to Apparent Power, or Watts to VA. Displacement power factor is the classic concept of power factor. It can be considered as the power factor at the fundamental frequency. Total Power Factor, abbreviated to Power Factor (PF), now includes the effects of fundamental and of harmonic currents (it is also referred to as True power factor or Distortion power factor, Below figure). It follows that with the presence of harmonics, power factor is always lower than displacement power factor and is also a more accurate description of total system efficiency than displacement power factor alone.
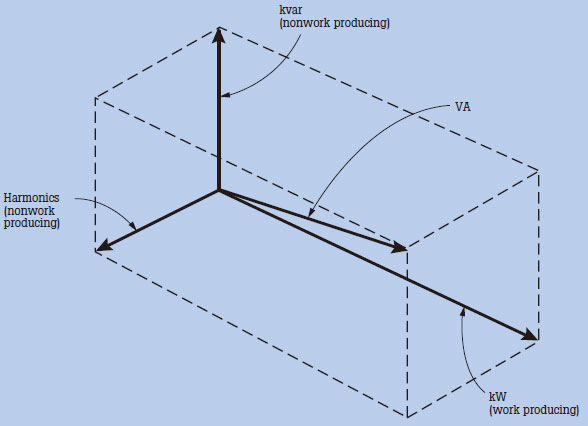
Strictly speaking, the term "Power Factor" refers to Total power factor, but in practice can also be used to refer to displacement power factor. Needless to say, this introduces some confusion into discussions of power factor. You have to be clear which one you’re talking about.
Displacement power factor
Lower displacement power factor is caused by motor loads which introduce the need for Reactive Power (Volt-Amp Reactive or VARs). The system has to have the capacity, measured in Volt-Amps (VA) to supply both VARs and Watts. The more VARs needed, the larger the VA requirement and the smaller the displacement power factor. The cost of VARs is accounted for in a power factor penalty charge. Utilities often levy additional charges for displacement power factor below a certain level; the actual number varies widely, but typical numbers are 0.90 to 0.95.
To reduce VARs caused by motor loads, power factor correction capacitors are installed. Upstream system capacity, both in the plant and at the utility level, is released and available for other uses.
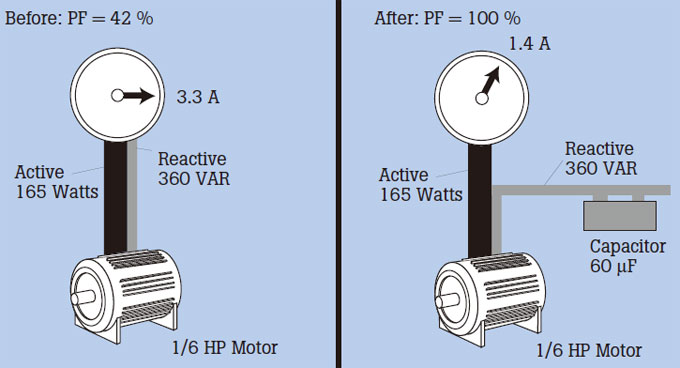
Historically, this has been the gist of the power factor story: a relatively well-known problem with a relatively straightforward solution.
Harmonics and capacitors
Harmonics have had a dramatic impact on our approach to Power Factor correction. The motor and capacitor loads described above are all linear and for all practical purposes generate no harmonics. Non-linear loads such as frequency inverter, on the other hand, do generate harmonic currents.
Take a plant which is step-by-step putting frequency inverters on its motor loads. Frequency inverters generate significant harmonic currents (5th and 7th on six pulse converter frequency inverters). Suddenly the fuses on existing power factor correction caps start blowing. Since these are three-phase caps, only one of the three fuses might blow. Now you’ve got unbalanced currents, possibly unbalanced voltages. The electrician replaces the fuses. They blow again. He puts in larger fuses. Now the fuses survive, but the capacitor blows. He replaces the capacitor. Same thing happens. What’s going on? Harmonics are higher frequency currents. The higher the frequency, the lower the impedance of a cap (XC = 1/2πfC). The cap acts like a sink for harmonic currents.
Power system resonance
In a worst-case scenario, the inductive reactance (XL) of the transformer and the capacitive reactance (XC) of the power factor correction cap form a parallel resonant circuit: XL = XC at a resonant frequency which is the same as or close to a harmonic frequency. The harmonic current generated by the load excites the circuit into oscillation. Currents then circulate within this circuit which are many times greater than the exciting current. This so-called "tank circuit" can severely damage equipment, and it will also cause a drop in power factor. Perversely, this resonant condition often appears only when the system is lightly loaded, because the damping effect of resistive loads is removed. In other words, we have what the audio buffs call a "high Q" circuit.
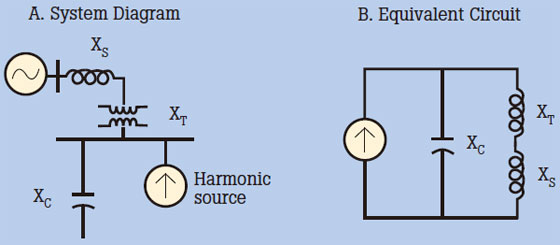
Imagine coming to work on a Monday and seeing the insulation on your cables melted off. How can this happen over a weekend when there was hardly any load on the system? Has Ohm's Law been overruled? Not quite. Your power system just spent the weekend tanked out on the Harmonics. It was quite a party, but now comes the clean-up.
Start with harmonics mitigation
The correct solution path starts with measuring and mitigating the harmonics generated by the frequency inverters. Harmonic trap filters would generally be called for. These trap filters are installed locally on the line side of the frequency inverter. Their effect is very much like the traditional power factor correction cap, in two senses: they reduce displacement power factor as well as power factor, and also they localize the circulation of the problem harmonics (generally the 5th). Harmonics mitigation and traditional displacement power factor correction should be addressed as one systems issue. In other words, manage Total power factor, not just displacement power factor.
Post a Comment:
You may also like: