Category
- Products
- FAQ
- Contact us
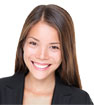
Frequency inverter manufacturers
What is Frequency Converter? How it works?
Variable frequency operation has been around, in the form of the AC generator, since the advent of the induction motor. Change the rotational speed of a generator and you change its output frequency. Before the advent of high speed transistors, this was one of the few options available to vary motor speed, however, frequency changes were limited because generator speed reduction lowered the output frequency but not the voltage.
We will see why this is important a little later. In our industry, variable speed pumping applications were far more complex in the past than they are today. One of the simpler methods was to employ a multi-pole motor that was wound in such a way that allowed a switch (or switches) to vary the number of stator poles that were active at any given time. Rotational speed could be changed manually or by a sensor connected to the switches. Many variable flow pumping applications still employ this method. Examples include hot & chilled water circulators, pool pumps, and cooling tower fans & pumps. Some domestic booster pumps used fluid drive or variable belt drive systems (an automatic transmission of sorts) to vary pump speed based on feedback from a pressure diaphragm valve. And, several others were even more complex.
Based upon the hoops we had to jump through in the past, it becomes pretty apparent why the advent of the modern frequency converter revolutionized (yet another pun) the variable speed pumping environment. All you have to do today is install a relatively simple electronic box (that often replaces more complex starting equipment) at the application site and, suddenly you can, either manually or automatically, change pump speed to your heart's desire.
So, lets take a look at the components of a frequency inverter and see how they actually function together to vary frequency and thus motor speed. I think you will be amazed at the simplicity of the process. All it took was the maturing of the solid state device we know as the transistor.
Frequency converter Components
The Rectifier
Because it is difficult to change the frequency of an AC sine wave while in the AC mode, the first job of a frequency converter is to convert the wave to DC. As you will see a little later, it is relatively easy to manipulate DC in order to make it look like AC. The first component of all frequency converters is a device known as a rectifier or converter and it is shown to the left of the figure below.
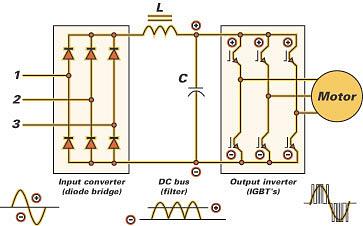
The rectifier circuit converts AC to DC and does so in much the same manner as those of a battery charger or arc welder. It uses a diode bridge to limit the travel of the AC sine wave to one direction only. The result is a fully rectified AC wave form that is interpreted by a DC circuit as a native DC wave form. Three phase frequency converters accept three separate AC input phases and convert them to a single DC output. Most three phase frequency converters can also accept single phase (230V or 460V) power but, since there are only two incoming legs, the frequency converters output (HP) must be derated because the DC current produced is reduced proportionally. On the other hand, true single phase frequency converters (those that control single phase motors) utilize a single phase input and produce a DC output that is proportional to the input.
There are two reasons why three phase motors are more popular than their single phase counter parts when it comes to variable speed operation. First they offer a much wider power range. But, equally as important is their ability to begin rotation on their own. A single phase motor, on the other hand, often requires some outside intervention to begin rotation. In this case, we will limit our discussion to three phase motors used on three phase frequency converters.
The DC Bus
The second component, known as the DC Bus (shown in the center of the illustration) is not seen and in all frequency converters because it does not contribute directly to variable frequency operation. But, it will always be there in high quality, general purpose frequency converters (those manufactured by dedicated frequency converter manufacturers). Without getting into a lot of detail, the DC Bus uses capacitors and an inductor to filter the AC "ripple" voltage from the converted DC before it enters the inverter section. It can also include filters which impede harmonic distortion that can feed back into the power source supplying the frequency converter. Older frequency converters and some pump specific frequency converters require separate line filters to accomplish this task.
The Inverter
To the right of the illustration is the "guts" of the frequency converter. The inverter uses three sets of high speed switching transistors to create DC "pulses" that emulate all three phases of the AC sine wave. These pulses not only dictate the voltage of the wave but also its frequency. The term inverter or inversion means "reversal" and simply refers to the up and down motion of the generated wave form. The modern frequency converter inverter uses a technique known as "Pulse Width Modulation" (PWM) to regulate voltage and frequency. We will cover this in more detail when we look at the output of the inverter.
Another term you have probably run across when reading frequency converter literature or advertisements is "IGBT". IGBT refers to the "Insulated Gate, Bipolar Transistor" which is the switching (or pulsing) component of the inverter. The transistor (which replaced the vacuum tube) serves two functions in our electronic world. It can act as an amplifier and increase a signal as it does in a radio or stereo or, it can act as a switch and simply turn a signal on and off. The IGBT is simply a modern version that provides higher switching speeds (3000 – 16000 Hz) and reduced heat generation. The higher switching speed results in increased accuracy of AC wave emulation and reduced audible motor noise. A reduction in generated heat means smaller heat sinks and thus a smaller frequency converter footprint.
Inverter Output
The illustration to the right shows the wave form generated by the inverter of a PWM frequency converter compared with that of a true AC sine wave. The inverter output consists of a series of rectangular pulses with a fixed height and adjustable width. In this particular case there are three sets of pulses -- a wide set in the middle and a narrow set at the beginning and end of both the positive and negative portions of the AC cycle. The sum of the areas of the pulses equals the effective voltage of a true AC wave (we will discuss effective voltage in a few minutes). If you were to chop off the portions of the pulses above (or below) the true AC wave and use them to fill in the blank spaces under the curve, you would find that they match almost perfectly. It is in this manner that a frequency converter controls the voltage going to the motor.
The sum of the width of the pulses and the blank spaces between them determines the frequency of the wave (hence PWM or pulse width modulation) seen by the motor. If the pulse was continuous (i.e. no blank spaces), the frequency would still be correct but the voltage would be much greater than that of the true AC sine wave. Depending upon the desired voltage and frequency, the frequency converter will vary the height and width of the pulse and the width of the blank spaces in between. Although the internals that accomplish this are relatively complex, the result is elegantly simple!
Now, some of you are probably wondering how this "fake" AC (actually DC) can run an AC induction motor. After all, doesn't it take an alternating current to "induce" a current and its corresponding magnetic field in the motor's rotor? Well, AC causes induction naturally because it is continuously changing direction. DC, on the other hand, does not because it is normally motionless once a circuit is activated. But, DC can induce a current if it is switched on and off. For those of you old enough to remember, automobile ignition systems (prior to the advent of the solid state ignition) used to have a set of points in the distributor. The purpose of the points was to "pulse" power from the battery into the coil (a transformer). This induced a charge in the coil which then increased the voltage to a level that would allow the spark plugs to fire. The wide DC pulses seen in the previous illustration are actually made up of hundreds of individual pulses and, it is this on and off motion of the inverter output that allows induction via DC to occur.
Effective Voltage
AC power is a rather complex quantity and it is no wonder that Edison almost won the battle to make DC the standard in the US. Fortunately, for us, all of its complexities have been explained and all we have to do is follow the rules those before us have laid out.
One of the attributes that makes AC complex is that it changes voltage continuously, going from zero to some maximum positive voltage, then back to zero, then to some maximum negative voltage, and then back to zero again. How is one to determine the actual voltage applied to a circuit? The illustration on the left is that of a 60 Hz, 120V sine wave. Notice, however, that its peak voltage is 170V. How can we possibly call this a 120V wave if its actual voltage is 170V? During one cycle it starts at 0V and rises to 170V then falls again to 0. It continues falling to –170 and then rises again to 0. It turns out that the area of the green rectangle, whose top border is at 120V, is equal to the sum of the areas under the positive and negative portions of the curve. Could 120V then be the average? Well, if you were to average all of the voltage values at each point across the cycle, the result would be approximately 108V so that must not be the answer. Why then is the value, as measured by a VOM, 120V? It has to do with something we call "effective voltage".
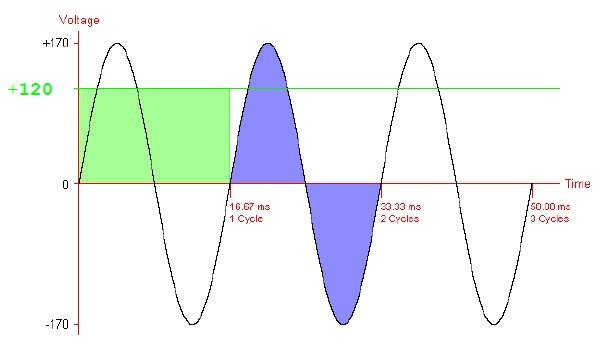
If you were to measure the heat produced by a DC current flowing through a resistance, you would find that it is greater than that produced by an equivalent AC current. This is due to the fact that AC does not maintain a constant value throughout its cycle. If you did this in the laboratory, under controlled conditions, and found that a particular DC current generated a heat rise of 100 deg, its AC equivalent would produce a 70.7 deg rise or just 70.7% of the DC value. Therefore the effective value of AC is 70.7% of that of DC. It also turns out that the effective value of an AC voltage is equal to the square root of the sum of the squares of the voltage across the first half of the curve. If the peak voltage is 1 and you were to measure each of the individual voltages from 0 deg to 180 deg, the effective voltage would be 0.707 of the peak voltage. 0.707 times the peak voltage of 170 seen in the illustration equals 120V. This effective voltage is also known as the root mean square or RMS voltage. It follows that the peak voltage will always be 1.414 that of the effective voltage. 230V AC current has a peak voltage of 325V while 460 has a peak voltage of 650V. We will see the effects of peak voltage a little later.
Well, I have probably gone on about this longer than necessary but, I wanted you to gain an understanding of effective voltage so that you will understand the illustration below. In addition to varying frequency, a frequency converter must also vary voltage even though voltage has nothing to do with the speed at which an AC motor operates.
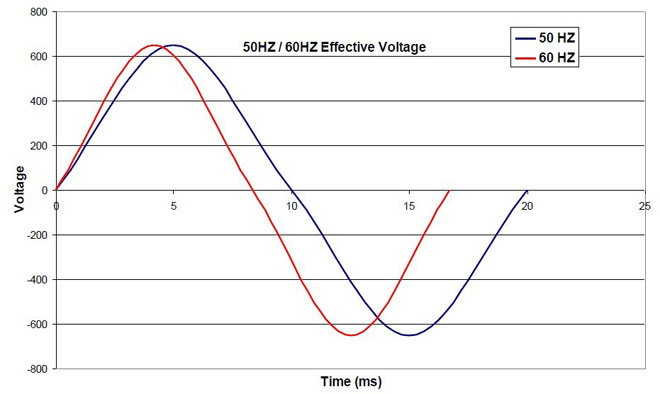
The illustration shows two 460V AC sine waves. The red one is a 60hz curve while the blue one is 50hz. Both have a peak voltage of 650V but, the 50hz is much broader. You can easily see that the area under the first half (0 – 10 ms) of the 50hz curve is greater than that of the first half (0 – 8.3 ms) of the 60hz curve. And, since the area under the curve is proportional to effective voltage, its effective voltage is higher. This increase in effective voltage becomes even more dramatic as frequency decreases. If a 460V motor were allowed to operate at these higher voltages, its life could be decreased substantially. Therefore, the frequency converter must constantly vary "peak" voltage, with respect to frequency, in order to maintain a constant effective voltage. The lower the operating frequency, the lower the peak voltage and vice versa. It is for this reason that 50hz motors, used in Europe and parts of Canada, are rated for 380V. See, I told you that AC can be a bit complex!
You should now have a pretty good understanding of the workings of a frequency converter and how it controls the speed of a motor. Most frequency converters offer the user the ability to set motor speed manually via a multi-position switch or keypad, or use sensors (pressure, flow, temperature, level etc) to automate the process.

Based upon the hoops we had to jump through in the past, it becomes pretty apparent why the advent of the modern frequency converter revolutionized (yet another pun) the variable speed pumping environment. All you have to do today is install a relatively simple electronic box (that often replaces more complex starting equipment) at the application site and, suddenly you can, either manually or automatically, change pump speed to your heart's desire.
So, lets take a look at the components of a frequency inverter and see how they actually function together to vary frequency and thus motor speed. I think you will be amazed at the simplicity of the process. All it took was the maturing of the solid state device we know as the transistor.
Frequency converter Components
The Rectifier
Because it is difficult to change the frequency of an AC sine wave while in the AC mode, the first job of a frequency converter is to convert the wave to DC. As you will see a little later, it is relatively easy to manipulate DC in order to make it look like AC. The first component of all frequency converters is a device known as a rectifier or converter and it is shown to the left of the figure below.
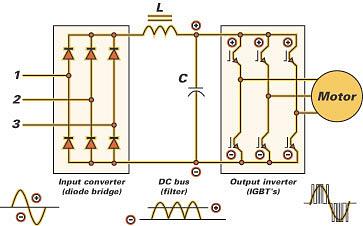
The rectifier circuit converts AC to DC and does so in much the same manner as those of a battery charger or arc welder. It uses a diode bridge to limit the travel of the AC sine wave to one direction only. The result is a fully rectified AC wave form that is interpreted by a DC circuit as a native DC wave form. Three phase frequency converters accept three separate AC input phases and convert them to a single DC output. Most three phase frequency converters can also accept single phase (230V or 460V) power but, since there are only two incoming legs, the frequency converters output (HP) must be derated because the DC current produced is reduced proportionally. On the other hand, true single phase frequency converters (those that control single phase motors) utilize a single phase input and produce a DC output that is proportional to the input.
There are two reasons why three phase motors are more popular than their single phase counter parts when it comes to variable speed operation. First they offer a much wider power range. But, equally as important is their ability to begin rotation on their own. A single phase motor, on the other hand, often requires some outside intervention to begin rotation. In this case, we will limit our discussion to three phase motors used on three phase frequency converters.
The DC Bus
The second component, known as the DC Bus (shown in the center of the illustration) is not seen and in all frequency converters because it does not contribute directly to variable frequency operation. But, it will always be there in high quality, general purpose frequency converters (those manufactured by dedicated frequency converter manufacturers). Without getting into a lot of detail, the DC Bus uses capacitors and an inductor to filter the AC "ripple" voltage from the converted DC before it enters the inverter section. It can also include filters which impede harmonic distortion that can feed back into the power source supplying the frequency converter. Older frequency converters and some pump specific frequency converters require separate line filters to accomplish this task.
The Inverter
To the right of the illustration is the "guts" of the frequency converter. The inverter uses three sets of high speed switching transistors to create DC "pulses" that emulate all three phases of the AC sine wave. These pulses not only dictate the voltage of the wave but also its frequency. The term inverter or inversion means "reversal" and simply refers to the up and down motion of the generated wave form. The modern frequency converter inverter uses a technique known as "Pulse Width Modulation" (PWM) to regulate voltage and frequency. We will cover this in more detail when we look at the output of the inverter.
Another term you have probably run across when reading frequency converter literature or advertisements is "IGBT". IGBT refers to the "Insulated Gate, Bipolar Transistor" which is the switching (or pulsing) component of the inverter. The transistor (which replaced the vacuum tube) serves two functions in our electronic world. It can act as an amplifier and increase a signal as it does in a radio or stereo or, it can act as a switch and simply turn a signal on and off. The IGBT is simply a modern version that provides higher switching speeds (3000 – 16000 Hz) and reduced heat generation. The higher switching speed results in increased accuracy of AC wave emulation and reduced audible motor noise. A reduction in generated heat means smaller heat sinks and thus a smaller frequency converter footprint.
Inverter Output
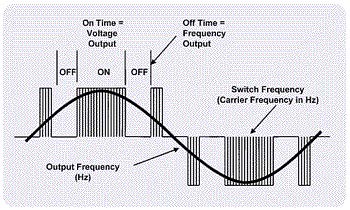
The sum of the width of the pulses and the blank spaces between them determines the frequency of the wave (hence PWM or pulse width modulation) seen by the motor. If the pulse was continuous (i.e. no blank spaces), the frequency would still be correct but the voltage would be much greater than that of the true AC sine wave. Depending upon the desired voltage and frequency, the frequency converter will vary the height and width of the pulse and the width of the blank spaces in between. Although the internals that accomplish this are relatively complex, the result is elegantly simple!
Now, some of you are probably wondering how this "fake" AC (actually DC) can run an AC induction motor. After all, doesn't it take an alternating current to "induce" a current and its corresponding magnetic field in the motor's rotor? Well, AC causes induction naturally because it is continuously changing direction. DC, on the other hand, does not because it is normally motionless once a circuit is activated. But, DC can induce a current if it is switched on and off. For those of you old enough to remember, automobile ignition systems (prior to the advent of the solid state ignition) used to have a set of points in the distributor. The purpose of the points was to "pulse" power from the battery into the coil (a transformer). This induced a charge in the coil which then increased the voltage to a level that would allow the spark plugs to fire. The wide DC pulses seen in the previous illustration are actually made up of hundreds of individual pulses and, it is this on and off motion of the inverter output that allows induction via DC to occur.
Effective Voltage
AC power is a rather complex quantity and it is no wonder that Edison almost won the battle to make DC the standard in the US. Fortunately, for us, all of its complexities have been explained and all we have to do is follow the rules those before us have laid out.
One of the attributes that makes AC complex is that it changes voltage continuously, going from zero to some maximum positive voltage, then back to zero, then to some maximum negative voltage, and then back to zero again. How is one to determine the actual voltage applied to a circuit? The illustration on the left is that of a 60 Hz, 120V sine wave. Notice, however, that its peak voltage is 170V. How can we possibly call this a 120V wave if its actual voltage is 170V? During one cycle it starts at 0V and rises to 170V then falls again to 0. It continues falling to –170 and then rises again to 0. It turns out that the area of the green rectangle, whose top border is at 120V, is equal to the sum of the areas under the positive and negative portions of the curve. Could 120V then be the average? Well, if you were to average all of the voltage values at each point across the cycle, the result would be approximately 108V so that must not be the answer. Why then is the value, as measured by a VOM, 120V? It has to do with something we call "effective voltage".
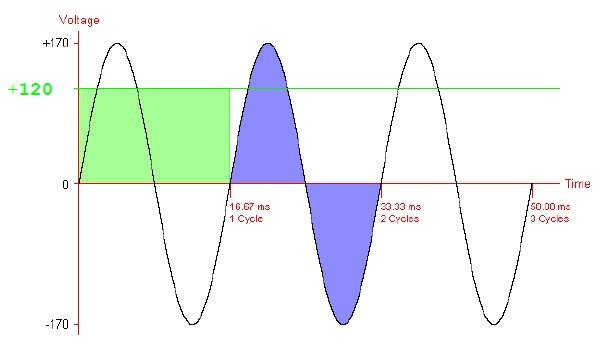
If you were to measure the heat produced by a DC current flowing through a resistance, you would find that it is greater than that produced by an equivalent AC current. This is due to the fact that AC does not maintain a constant value throughout its cycle. If you did this in the laboratory, under controlled conditions, and found that a particular DC current generated a heat rise of 100 deg, its AC equivalent would produce a 70.7 deg rise or just 70.7% of the DC value. Therefore the effective value of AC is 70.7% of that of DC. It also turns out that the effective value of an AC voltage is equal to the square root of the sum of the squares of the voltage across the first half of the curve. If the peak voltage is 1 and you were to measure each of the individual voltages from 0 deg to 180 deg, the effective voltage would be 0.707 of the peak voltage. 0.707 times the peak voltage of 170 seen in the illustration equals 120V. This effective voltage is also known as the root mean square or RMS voltage. It follows that the peak voltage will always be 1.414 that of the effective voltage. 230V AC current has a peak voltage of 325V while 460 has a peak voltage of 650V. We will see the effects of peak voltage a little later.
Well, I have probably gone on about this longer than necessary but, I wanted you to gain an understanding of effective voltage so that you will understand the illustration below. In addition to varying frequency, a frequency converter must also vary voltage even though voltage has nothing to do with the speed at which an AC motor operates.
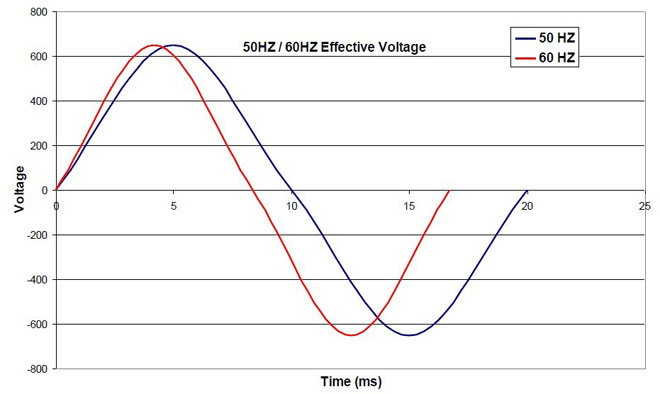
The illustration shows two 460V AC sine waves. The red one is a 60hz curve while the blue one is 50hz. Both have a peak voltage of 650V but, the 50hz is much broader. You can easily see that the area under the first half (0 – 10 ms) of the 50hz curve is greater than that of the first half (0 – 8.3 ms) of the 60hz curve. And, since the area under the curve is proportional to effective voltage, its effective voltage is higher. This increase in effective voltage becomes even more dramatic as frequency decreases. If a 460V motor were allowed to operate at these higher voltages, its life could be decreased substantially. Therefore, the frequency converter must constantly vary "peak" voltage, with respect to frequency, in order to maintain a constant effective voltage. The lower the operating frequency, the lower the peak voltage and vice versa. It is for this reason that 50hz motors, used in Europe and parts of Canada, are rated for 380V. See, I told you that AC can be a bit complex!
You should now have a pretty good understanding of the workings of a frequency converter and how it controls the speed of a motor. Most frequency converters offer the user the ability to set motor speed manually via a multi-position switch or keypad, or use sensors (pressure, flow, temperature, level etc) to automate the process.
Excellent article and very good explanation of how frequency convertors control AC induction motor speed.
Can we use the same inverter for 1400cycle and 2800cycle a.c. motors?
Thank you for the very informative article!
That is a good share of knowledge and excellent article.9
clear explanation, thank you!
Thank you for the very informative article!
Excellent article and very good explanation of how frequency convertors control AC induction motor speed.
Excellent article and very good explanation of how frequency convertors control AC induction motor speed.
Very nice....thank you
Post a Comment:
You may also like:
i have a project in my college about building static inverter (input:3 phase 220V (50 hz) output: 3 phase 115V (400hz) with 20Ampere
if your company help me you glad me very much
please help me please
i don't need a beforehand product i just need circuit scheme to build it myself